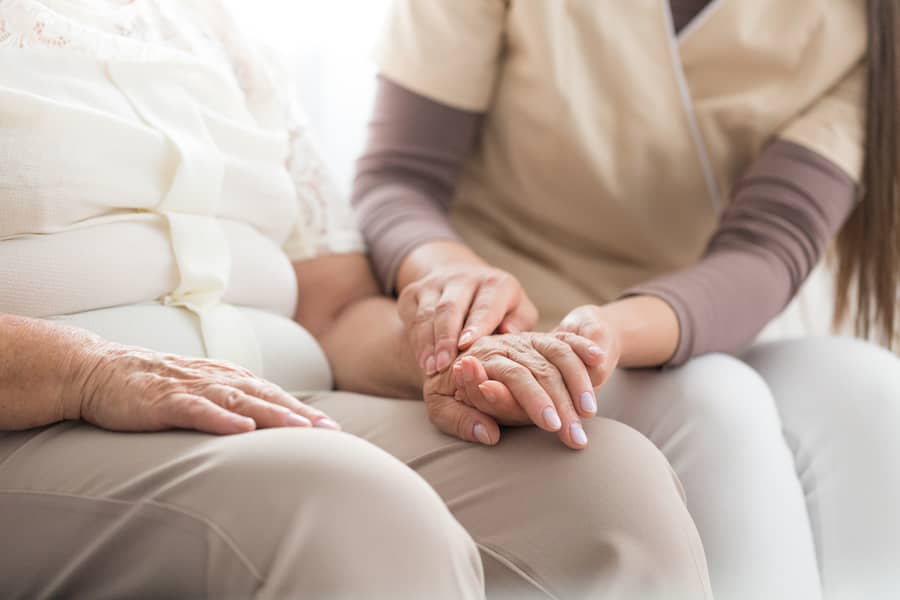
Deep brain stimulation (DBS), a neurosurgical treatment that stimulates the brain with electrical signals, is used to treat Parkinson’s disease (PD) and an ever-increasing list of neurological disorders. Despite growing numbers of applications, DBS is at a relative technological standstill due to several factors: limited choice of stimulus waveforms, ability to stimulate at only a single location and inefficient use of battery power. The only allowable input is a periodic train of square pulses applied in an extremely small region of the brain (a few mm). Although some degree of customization is allowed (e.g., change of pulse width, frequency, amplitude), the resulting signals cannot generate a wide variety of controlled responses from the targeted neural system, limiting the patient’s behaviors. It is likely that the therapeutic impact of such constant periodic stimulation comes from replacing the pathological rhythmic basal ganglia (BG) output seen in PD with tonic, high frequency (HF) firing. This increased activity prevents neurons from modulating activity in their neighboring structures creating an “information lesion” in the area. HF stimulation provides clinical benefits (PD motor symptoms reversal) when the targeted area is highly pathological but comes with significant costs: lengthy manual programming of the signal, no adaptation to patient’s needs, frequent surgical battery replacements, and widespread influence to nearby cognitive loops with possible adverse side effects.
There is evidence that non-Parkinsonian neural activity is irregular and much lower in frequency. Therefore, investigators have attempted to restore neural activity and address the shortcomings of HF DBS using alternative low frequency and irregular stimulation patterns, but poor clinical results have been reported. These poor results stem from both technology limitations of fixed stimulation in a single location and lack of understanding of the neural circuit being stimulated. New DBS strategies (different and/or multiple sites and patterns) for PD could be evaluated if we had a deeper understanding of the dynamical interactions between nuclei in the cortico-basal ganglia-thalamic loop in normal and in PD, with and without DBS.
We are developing a novel systems modeling framework for studying the dynamics of the BG-cortical loop. In order to develop this modeling framework, we will exploit a unique experimental set up (microelectrode single-unit and local field potential (LFP) recordings from the BG and sensory/motor cortex in nonhuman primates, before and during DBS), and characterize the input-output relationship of BG nuclei and cortex, with and without DBS. Our research will extend preliminary work, wherein we successfully used point process models (PPMs) to characterize spike train dynamics in the subthalamic nucleus (STN), striatum, sensorimotor cortex, and Globus Pallidus internus (GPi) in the BG.